Southern Sweden is home to both MAX IV, an X-ray facility open since 2016, and the European Spallation Source (ESS), a neutron facility set to open for users in 2027, which make up two thirds of the large-scale research infrastructures that InfraLife represents. We interviewed Derek Logan (Professor of Biochemistry and Structural Biology, Lund University (LU)) about how he has used both X-rays and neutrons to investigate galectin-3, an important protein in health and disease.
X-ray macromolecular crystallography provided the starting point for determining the structure of galectin-3
Galectins are small, soluble proteins which are implicated in multiple diseases such as inflammation, diabetes and cancer (Logan et al., 2019). This makes them an ideal target for drug design but unfortunately due to the exposed, polar nature of their binding sites, it is difficult to achieve. The I911-5 beamline at MAX-II Lab, one of MAX IV’s predecessors, was used to determine the protein crystal structure of galectin-3 to a record high resolution at the time of 0.86 Å (Saraboji et al., 2012) using X-ray macromolecular crystallography. An important feature of galectins is their binding sites which recognize sugar molecules on the cell surface of proteins, thus enabling binding and potential inhibitor interaction. These binding sites involve hydrogen bonds which are not detectable by X-rays, and hence where the need for neutron experiments arose. All successful galectin inhibitors developed to date have a central sugar component. A collaboration with the organic chemists at the Centre for Analysis at Synthesis (CAS, LU) began in order to determine how important the sugar component was, if the direction of the hydrogen bonds played a role and lastly, if you could replace the hydrogen bonding atoms with other atoms in new inhibitors against galectin.
Neutron crystallography allows for the identification of hydrogen bonds; an important feature in ligand-inhibitor binding
An advantage with neutrons is their ability to detect lighter elements such as hydrogen. A common technique used to observe hydrogens is by replacing them with deuterium (“heavy hydrogen”) in a process called perdeuteration, which enables better contrast within the samples. Despite having the relevant expertise within their research group, using a dedicated facility to produce deuterated proteins is easier, more economical and allows for reuse of expensive reagents by the facility. In this case, Lund Protein Production Platform (LP3) was used, which now has the Deuteration and Macromolecular Crystallisation (DEMAX) of ESS located at the same facility. Initial neutron macromolecular crystallography was conducted at Los Alamos National Laboratory but unfortunately the neutron beam was not strong enough for the relatively small crystals, and later successful experiments were conducted at the Institut Laue-Langevin (ILL, France). Due to the non-destructive nature of neutrons, it was possible to use the same crystals at both facilities. Neutron crystal structures were determined for both the unbound and bound galectin-3C providing the positions of key hydrogen atoms which are involved in ligand binding for the first time experimentally (Manzoni at el., 2018). Furthermore, the hydrogen bonding did indeed display directional specificity, confirming the mechanism of action of current galectin-3 inhibitors, but allowed more options for future inhibitors which could take advantage of the now identified differences in hydrogen bonding between water molecules and ligands.
Combining X-ray and neutron data with computational chemistry allows for a more detailed and full refinement of the protein structure
A large part of this research was conducted by a PhD student, Francesco Manzoni, who worked with both Prof. Logan’s group and Ulf Ryde, Professor in the Division of Computational Chemistry, LU and was partially funded by ESS. This shared position allowed the use of quantum-mechanical calculations in combination with X-ray and neutron crystallographic data of galectin-3 to fully refine the protein structure (Caldararu et al., 2019), leading to better-resolved hydrogen-bonding interactions with the water molecules.
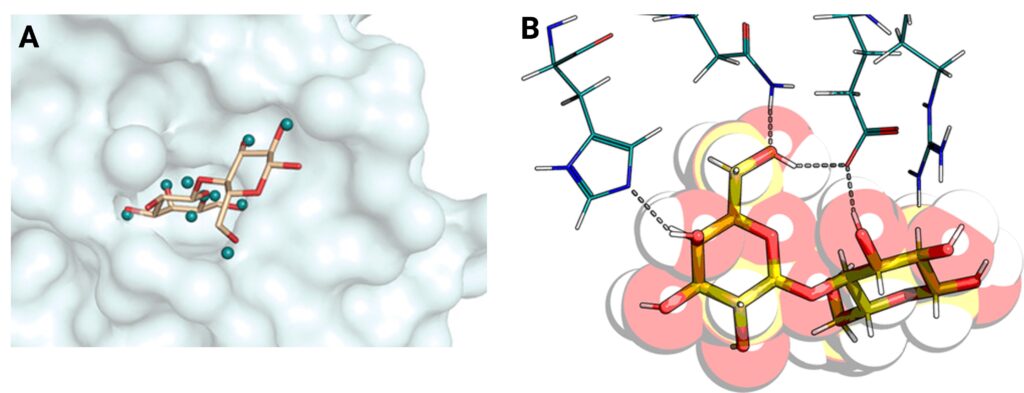
The future possibilities in Sweden for structural biology using X-rays and neutrons
As described here, using both X-rays and neutrons are complimentary and oftentimes both techniques are required to obtain full refinement of a structure. It is also not possible to determine a structure with neutrons alone. MAX IV has two beamlines for macromolecular crystallography, BioMAX and MicroMAX, in addition to the FragMAX platform which enables fragment-screening for potential drug discovery. The NMX instrument at ESS is a macromolecular diffractometer which will allow for the determination of protein structures faster and with smaller crystals, thus enabling more challenging structural biology projects and reduced sample preparation and data collection times. ESS also has a dedicated Data Management and Software Centre (DMSC) located in Denmark, which will provide services and solutions to ESS users. Conducting neutron experiments first and then X-rays is the desirable route, but this is not always possible. In 2027 when both ESS and MAX IV are in operation for users it should enable more of these types of experiments. Having a close collaboration between these facilities and perhaps having overlapping proposals for access could further enable these experiments. Prof. Logan points out the difficulties associated with the logisitics of transporting crystals for neutron experiments, from airport security to movement of the crystals within the transport capillary. Another major difference between using X-rays and neutrons for determining protein structures is the size of crystals needed, with neutrons requiring much larger ones. This can often be a limiting factor. Given this lengthy time to produce them, having the option to use a local facility like ESS and remove these issues, is very appealing. Lastly, the emergence of a new Cryo-Electron Microcopy (Cryo-EM) at MAX IV is set to open for users in 2025 and opens new possibilities for synergies within structural biology.
Further information
Contact information
If you like to know more about how SciLifeLab, MAX IV and ESS can help with your Life Science needs, reach out to the Project Coordinators (Claire Lyons, claire.lyons@maxiv.lu.se or Josefin Lundgren Gawell, josefin.lundgren.gawell@scilifelab.se).
For more information about the galectin 3 research, please read the featured publications below or contact Derek Logan (derek.logan@biochemistry.lu.se).
References
Derek T. Logan. Hunting down hydrogen: applying neutron macromolecular crystallography to galectins. The Biochemist (Lond) 2019, 41 (2), 20-23. https://portlandpress.com/biochemist/article/41/2/20/1516/Hunting-down-hydrogen-applying-neutron
Kadhirvel Saraboji, Maria Håkansson, Samuel Genheden, Carl Diehl, Johan Qvist, Ulrich Weininger, Ulf J. Nilsson, Hakon Leffler, Ulf Ryde, Mikael Akke and Derek T. Logan. The Carbohydrate-Binding Site in Galectin-3 Is Preorganized To Recognize a Sugarlike Framework of Oxygens: Ultra-High-Resolution Structures and Water Dynamics. Biochemistry 2012, 51 (1), 296-306. https://pubs.acs.org/doi/10.1021/bi201459p
Francesco Manzoni, Johan Wallerstein, Tobias E. Schrader, Andreas Ostermann, Leighton Coates, Mikael Akke, Matthew P. Blakeley, Esko Oksanen, and Derek T. Logan. Elucidation of Hydrogen Bonding Patterns in Ligand-Free, Lactose- and Glycerol-Bound Galectin-3C by Neutron Crystallography to Guide Drug Design. Journal of Medicinal Chemistry 2018, 61 (10), 4412-4420. https://pubs.acs.org/doi/10.1021/acs.jmedchem.8b00081
Octav Caldararu, Francesco Manzoni, Esko Oksanen, Derek T. Logan and Ulf Ryde. Refinement of protein structures using a combination of quantum-mechanical calculations with neutron and X-ray crystallographic data. Acta Cryst. D75 2019, 75 (4), 368-380. https://journals.iucr.org/d/issues/2019/04/00/ei5038/index.html
Are you interested in using a research infrastructure, but not sure where to go or who to talk to?